(Dr. Leyla Moghaddasi, New South Wales, Australia)
Neutrons in Action: Neutron Stars
ONE OF NATURE’S MOST WONDROUS CREATIONS
In the field of medical physics, there is an air of mystery and bitter-sweet feeling about neutrons. We know of neutrons, fast or epithermal, as a highly effective form of ionising radiation in radiotherapy. Contrarily, they present as nuisance by-products of high megavoltage photon beams and are handled as contamination.
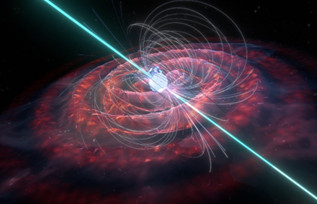
Beyond the realm of medical physics, however, neutrons are quite a spectacle! Matter in any state consists largely of empty space. The reason is matter is constituted of atoms made of clouds of electrons orbiting the nucleus. Nuclei make up more than 99.9% of atom mass, while their radius is on average 105 times less than electron clouds’ radius. When the core of a massive star undergoes gravitational collapse at the end of its life, atoms are crushed completely, and the electrons are scrunched inside the protons to form a star composed almost entirely of neutrons, leaving behind a neutron star. A tiny star composed of a gigantic nucleus and no empty space.
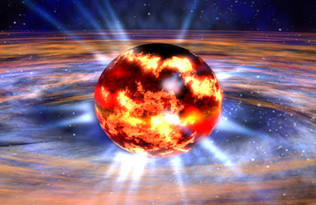
“With neutron stars, we’re seeing a combination of strong gravity, powerful magnetic and electric fields, and high velocities,” says the Large Area Telescope (LAT) science team of NASA’s Goddard Space Flight Centre in Greenbelt. The Crab Nebula; Credit: NASA.
The most known type of neutron stars are pulsars which are classified into rotation-powered, and accretion powered pulsars. Rotation-powered pulsars, such as the Crab Nebula, are relatively young neutron stars that rotate extremely rapidly. As a result, their strong magnetic fields combined with rapid rotation create magnetic fields trillions of times stronger than Earth’s. Pulsars’ magnetic fields are practically high-energy particle accelerators that can create deadly blizzards of high-energy particles.
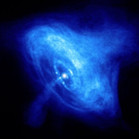
Accretion-powered pulsars, exhibiting behaviours much like Black Holes, are produced when neutron stars in binary star systems capture matter from a companion star. The captured matter forms a disk around the neutron star. The powerful magnetic field of the neutron star funnels the matter towards the poles where it will spiral down and fall, or accrete, onto the neutron star. The infalling matter will gain an enormous amount of energy as it accelerates. Most of this energy will be radiated away at X-ray energies, the phenomenon that gives them the name the Universe Light House. Now you may wonder why a neutral (uncharged) particle could possess a strong magnetic field. The short answer is the spin of neutrons. Particles with spin can possess a magnetic dipole moment, just like a rotating electrically charged body in classical electrodynamics. Let us have a quick peek into Particle Physics to understand where neutrons inherit their magnetic dipoles.
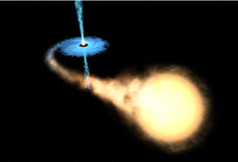
In the standard model of particle physics, particles are composed of elementary particles that are subatomic particles not composed of other particles. All elementary particles are currently thought to be either bosons or fermions. Their spin is differentiated via the spin-statistics theorem: it is a half-integer for fermions and an integer for bosons. Elementary fermions include leptons, antileptons, quarks and anti-quarks which generally are matter particles and antimatter particles. Elementary bosons (gauge bosons and the Higgs boson) act as force carriers that mediate interactions among fermions.
Quarks, classified into six types/flavours (up, down, charm, strange, top, and bottom) have various intrinsic properties, including non-integer electric charge, mass, colour charge, and spin. Unlike other elementary particles, they experience all fundamental forces, i.e., electromagnetism, gravitation, strong interaction, and weak interaction. Quarks are combined to form hadrons, with neutrons and protons being the most stable. In the quark model for hadrons, the neutron is composed of one up quark (charge +2/3 e) and two down quarks (charge – 1/3 e), resulting in a non-charged fermionic particle, the hero of this story.