Go to AFOMP Pulse Contents/Index Page
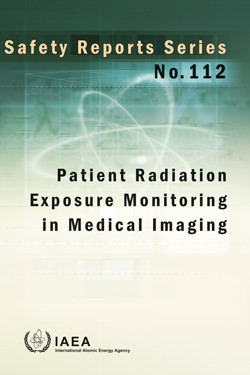
About IAEA Report Series 112
The expanded utilization of ionizing radiation in medical imaging has resulted in a notable increase in collective doses, highlighting the necessity for heightened oversight to ensure patient radiation protection. Despite the justified benefits of increased imaging, concerns arise from reports of unjustified and unoptimized use, often due to limited training in radiation protection among medical professionals and the growing complexity of imaging technologies. IAEA standards, such as the Fundamental Safety Principles and International Basic Safety Standards, establish requirements for patient dosimetry and diagnostic reference levels (DRLs), crucial for optimizing radiation protection. However, gaps persist in implementing these standards, prompting efforts to enhance exposure monitoring and communication, as evidenced by IAEA Technical Meetings.
As monitoring patient radiation exposure is vital for continuously improving radiation protection and patient care, IAEA published report series number 112 on ‘Patient Radiation Exposure Monitoring in Medical Imaging’. The publication provides comprehensive guidance on implementing monitoring systems, addressing metrics characterizing patient exposure, mechanisms for data collection and analysis, and practical implementation considerations. It emphasizes the significance of systematic monitoring, whether manual or digital, with a preference for automatic digital systems due to their effectiveness. The guidance covers diagnostic radiology, interventional procedures, and nuclear medicine, with an emphasis on ensuring meaningful access to exposure data. While offering expert opinions on good practices, the publication acknowledges that specific recommendations may vary across imaging modalities and does not represent a consensus of Member States.
Inside the report
Exposure metrics stem from both image acquisition parameters and patient characteristics. Fundamentally, monitoring patient exposure is justified by its direct impact on patient well-being, as the entire process aims to identify and mitigate radiation risks to the patient. Ideally, the focus should be on measuring and managing patient risk directly. However, individual patient risk is often uncertain or inaccessible. As an alternative, various proxies, ranging from modality-specific measures to more patient-centred ones, are employed. Modality-specific metrics are typically easier to determine and assign to an imaging procedure. Yet, their relevance lies in their ability to be more closely linked to patient exposure. The guideline hence outline the exposure metrics spanning from modality-specific to patient-oriented perspectives.
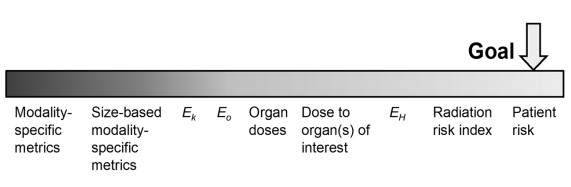
Figure 1.The spectrum of patient exposure metrics, ranging from modality-specific (left) to patient orientated (right) surrogates, shown by relevance hierarchy in terms of how well they can be related to the risk of the individual patient. E values represent various methods of calculating the effective dose: Ek is calculated from modality-specific standard conversion factors for a generic reference person: E0 uses organ doses calculated for a generic reference person; and EH uses organ doses calculated based on the anatomical definition of the actual patient
Evaluating imaging based on image quality establishes a quantitative basis for appropriate exposure levels in which image quality metrics aid in this assessment. While metrics related to phantoms and specific modalities, as been shown in Figure 2, are easier to determine, their relevance lies in their correlation with clinical quality. In assessing imaging studies, it’s essential to recognize the variability in quantities across multiple series or views. While exposure values can be summed to determine total exposure, this isn’t feasible for image quality values. Balancing image quality and exposure must be done at the individual series level, often utilizing data-informed mathematical analyses for studies with multiple series or views.
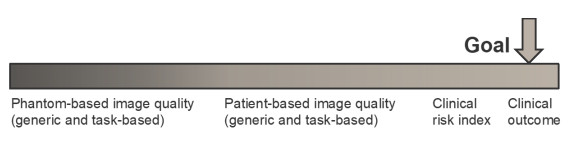
Figure 2.The spectrum of image quality metrics, ranging from phantom based, modality-specific surrogates (left) to patient orientated surrogates (right), shown by relevance hierarchy in terms of how well the quality of the images relates to a definitive clinical outcome for the patient.
The primary metrics of physical image quality, including resolution, contrast, and noise, are often derived from phantom-based measurements. However, these metrics offer limited insight into how specific aspects of image quality might affect diagnostic processes. While phantom measurements align well with certain attributes in clinical images, such as patient size, they fail to capture the variability present in patients’ characteristics. Furthermore, modern imaging systems adapt to individual patient attributes, yet these adaptations may not fully account for variations across patient data.
Image quality assessment can be approached through generic or task-based methods. Generic methods, akin to phantom-based metrics, lack specificity to particular tasks and rely on subjective preference studies by radiologists. In contrast, task-based assessments involve clinicians evaluating images based on specific imaging tasks, but are prone to significant variability between cases and observers, making statistical improvement challenging. Recent advancements enable the direct measurement of image quality from individual patient images, both in generic and task-specific contexts, without observer interpretation. Image quality measurement is crucial as it aims to ensure the clinical information provided is of high quality, thus mitigating the risk of suboptimal clinical outcomes. Similar to the radiation risk index, a clinical risk index reflects the actual clinical quality of an image for its intended purpose.
In addition, this report also underscored the importance of monitoring patient radiation exposure, alongside assessing patient exposure and image quality metrics. Monitoring patient radiation exposure involves several steps, the extent of which depends on available resources. These steps, depicted in Figure 3 being detailed further in this report, require systematic examination classification and coding systems to ensure consistent and comparable data. Recording patient exposure data in medical imaging entails documenting this information either manually or automatically, with modern digital X-ray tools automatically exporting radiation exposure details in DICOM format, capturing each irradiation event separately, such as CT scans or fluoroscopy sessions. Typically, a DICOM Radiation Dose Structured Report (RDSR) summarizes all events and includes patient demographics, study details, imaging technique, and dose metrics. Some systems output dose values in non-DICOM formats, which can still be archived and integrated using optical character recognition. However, systems lacking DICOM or non-DICOM outputs may require manual recording. Collecting this data involves aggregating it into a unified system, with data collected based on various schemes reflecting the collection’s purpose, such as specific facilities or examination types, and stored according to analysis objectives, often classified into multiple categories. Collection methods can be digital or manual, occurring in real-time or at intervals, as needed.
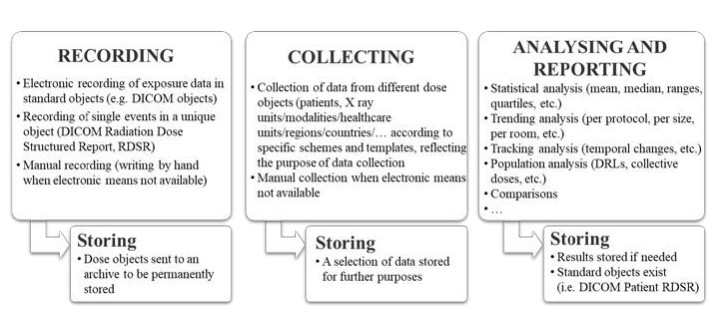
Figure 3. Steps in the process of radiation exposure monitoring.
Conclusions
Reviews of patients’ radiation exposure in medical imaging are essential tools for optimizing radiation protection, analysing individual and population-based exposures, and justifying procedures. This is particularly informative for assessing trends in collective doses and supporting epidemiological studies on radiation effects. Technological advancements in medical imaging have enhanced access to patient exposure data, fostering analytical uses. The purpose of this publication is to address the lack of definitive guidelines and offer consolidated information on monitoring patient radiation exposure. It emphasizes the importance of automatic digital systems due to the ease of accessing digital data. The hope is that this guideline will encourage the future development and use of such systems, contributing to the improved implementation of patient radiation protection worldwide.