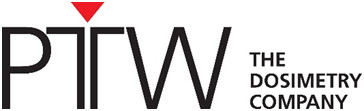
Imagine your department has just purchased a new state-of-the-art water phantom. It is standing right in front of you in the dimly lit linac room, ready and eager to perform measurements. You start with a challenging task – beam profile measurements of small fields. And you know what you require for this task: a small-size detector with minimal perturbation, fast measurement speed, and known small-field correction factors. However, even though you know which properties you need, the wide range of available detector models makes it somewhat overwhelming to determine the best detector for your task.
To simplify the selection process, it is essential to understand
- the key properties of a detector and how they impact performance
- the detector properties important for your specific application
- and the minimum requirements for each property
The following key properties can help you identify the most suitable detector for your task. The list is comprised of “hard” criteria, e.g., major performance characteristics, as well as “soft” criteria such as ease of use.
Key properties to consider when choosing a detector
Availability of background knowledge
Each detector has its own characteristics and behaves differently depending on the application. Understanding the performance characteristics of a detector, such as dose rate dependence, temperature and energy dependence, polarity or pre-irradiation, is crucial for accurate dosimetry. To do this, check if the manufacturer provides detailed specifications or access scientific publications for more insights and compare this information against relevant standards. PTW provides comprehensive technical specifications for each detector, as well as educational resources through lectures and webinars.
Availability of reliable correction factors
For applications such as reference dosimetry or small-field output measurements, it is essential to apply correction factors for accurate results. Evaluate the source and quality level of the correction factors to ensure accuracy and reliability of your dose measurements.
Range of use
The range of use for a detector is determined through extensive testing in accordance with international standards such as IEC 607311. It defines the approved operating conditions under which a detector can be reliably used. It is important to only use a detector within its specified range of use to minimize uncertainties. Operating a detector outside of its specified range of use can lead to increased uncertainties. By strictly adhering to the validated range of use, you can guarantee the reliability and accuracy of your measurements.
The PTW microDiamond detector is an example of a detector that offers a wide range of use as shown in Figure 1. As a versatile detector, it can be effectively used for different radiation qualities and for measurements from (very) small to large field sizes
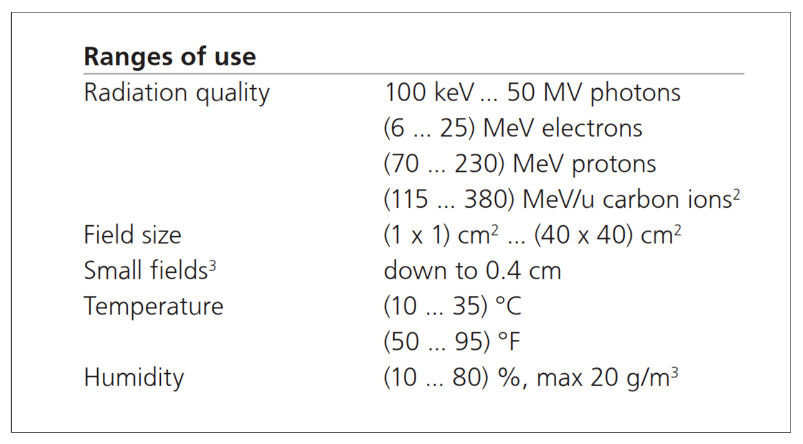
Ease of use
A detector that is easy to use reduces the risk of measurement errors significantly. A directly readable signal, for example, makes a detector easy to use. Having as few processing steps as possible is also important for reliable and reproducible operation.
Fast measurement or speed of scanning
When you schedule a measurement session, you will notice that a considerable amount of time is spent on the measurements themselves. The faster your detector can move in the water phantom – while maintaining signal quality – the faster your measurements are completed. The speed of measurement relates directly to the relative noise of a detector. Solid-state as well as scintillation detectors are far more noisy than air-filled ionization chambers. As a rule of thumb, the lower the noise, the faster you can measure.
Necessary pre-irradiation
Some detectors require pre-irradiation to stabilize their response. Before the pre-irradiation dose is reached, the response of such a detector will drift and change slightly. Other detectors need not be pre-irradiated and can start measuring right away. Understand if and how much pre-irradiation is needed for your detector to ensure accurate measurements.
Long-term stability
The long-term stability refers to the extent to which the calibration coefficient of a detector may change per year for a specific detector model. This property is crucial for detectors used for reference dosimetry.
Polarity dependence
When a detector is irradiated with a positive or negative bias, the resulting absolute value of the signal will vary slightly. The reasons for this are detector effects which (i) lead to a measurable signal and (ii) are independent of the applied bias. The polarity effect can differ in both magnitude and sign depending not only on the detector model, but also on the measurement situation. Utilizing a detector with minimal polarity dependence reduces uncertainty. Polarity corrections require different formulas for relative dosimetry and reference dosimetry. While averaging both signals is standard practice for relative dosimetry, a specialized correction formula is required for reference dosimetry. This formula is detailed in the “Code of Practice” section of the PTW DETECTORS catalogue.
Energy response
Water-equivalent detectors exhibit a good energy response and accurately measure dose to water in various positions within a water phantom. They perform well both within and outside of the primary beam, and their signal is independent of the measurement depth. In contrast, the signal of a detector with poor energy response depends on its position within the water phantom, or to be precise, it depends on the spectrum of the radiation at that position. For instance, a pure silicon detector may over-respond when deep in water, especially when measuring large fields or used outside of the beam. To avoid this problem, special silicon detectors are available that shield the sensitive volume against low-energy radiation.
Temperature dependence
You may have noticed that many things are temperature dependent. Your car may not start at freezing temperatures, or you may be less motivated to go jogging when outside temperatures drop. The same applies to detectors: the amount of collected charges varies with temperature, usually within a range of 0 and 0.6 %/K. This information can be found in the technical specifications of the detector.
Small-field effects (density & volume)
When planning to use a detector for small-field measurements, you should know its small-field properties. The most important properties are the volume2 and mass density of the detector. Knowing the correction factors for any detector used in small fields is crucial for effective operation.
Dose-rate dependence
The response of a detector may vary slightly depending on the dose rate or dose per pulse of the radiation. This effect is fairly weak, amounting to less than 1.5 % in most practical situations. However, the effect is more pronounced for IORT electron linacs, FLASH dose rates, liquid-filled detectors, or broken detectors. Similar to temperature dependence, dose-rate dependence is a property that should be taken into account when estimating uncertainty.
Energy response Water-equivalent detectors exhibit a good energy response and accurately measure dose to water in various positions within a water phantom. They perform well both within and outside of the primary beam, and their signal is independent of the measurement depth. In contrast, the signal of a detector with poor energy response depends on its position within the water phantom, or to be precise, it depends on the spectrum of the radiation at that position. For instance, a pure silicon detector may over-respond when deep in water, especially when measuring large fields or used outside of the beam. To avoid this problem, special silicon detectors are available that shield the sensitive volume against low-energy radiation.
Stability
Not all chambers are equal. While this may sound like a quote from the book Animal Farm, it actually refers to a detector property. The kQ value, necessary for reference dosimetry, is now calculated using an ideal Monte Carlo model of a detector. However, your measurement detector has been produced mechanically, and will have some production tolerances. The more your detector equals the ideal Monte Carlo model, the more accurate the kQvalue will be for your individual detector. A possible measure for production reproducibility is the variation of the calibration coefficient over the serial number of a specific detector type (see B. R. Muir, Med. Phys. 42, pp. 1546, 2015 for an example).
Application-centered detector choice
Understanding the key properties outlined above aids in selecting the most suitable detector for your specific measurement task. We recommend adopting an application-centered approach in making your decision. Start by choosing your application, then review the list of key properties to determine which are crucial for your application. This method will help narrow down your choices and lead you to the optimal detector for your task. Figures 2 and 3 contain charts that can guide you in selecting the best detector for reference and relative dosimetry applications.
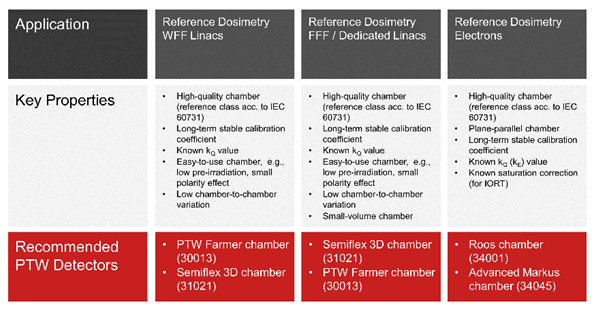
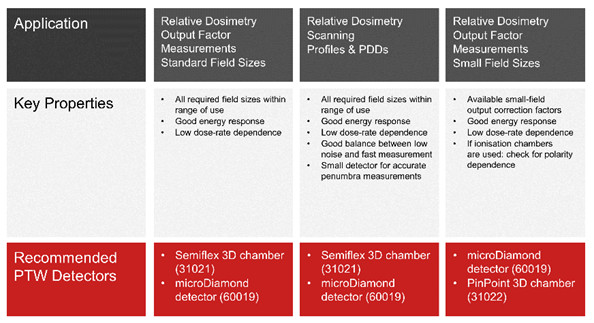
For more information on the properties and use of PTW detectors for specific applications, download the PTW Detectors Catalogue (with Code of Practice) and Small Field Application Guide, or check out the Detector Selector on the PTW website.
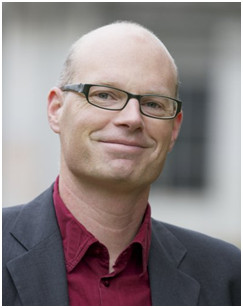
Jan Würfel studied physics at Karlsruhe Institute of Technology (KIT) and holds a Ph.D. in molecular electronics. He works as a research scientist at PTW Freiburg, focusing on the development of dosimetry equipment and detector physics. In addition, Jan serves as a speaker at international conferences and is involved in national and international standardization of dosimetry.
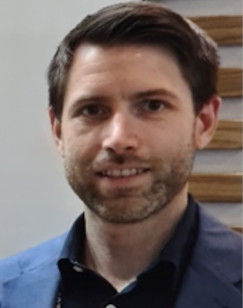
Konstantin Burzlaff holds a Master’s degree in Medical Engineering and currently works as a product manager at PTW Freiburg. In this role, he is responsible for expanding and further developing new dosimeter solutions for reference and relative dosimetry in radiotherapy.
[1] IEC 60731 Medical electrical equipment – Dosimeters with ionization chambers as used in radiotherapy
[2] To be precise, what actually counts is not the active volume of the detector, but the cross-section of the active volume perpendicular to beam direction.